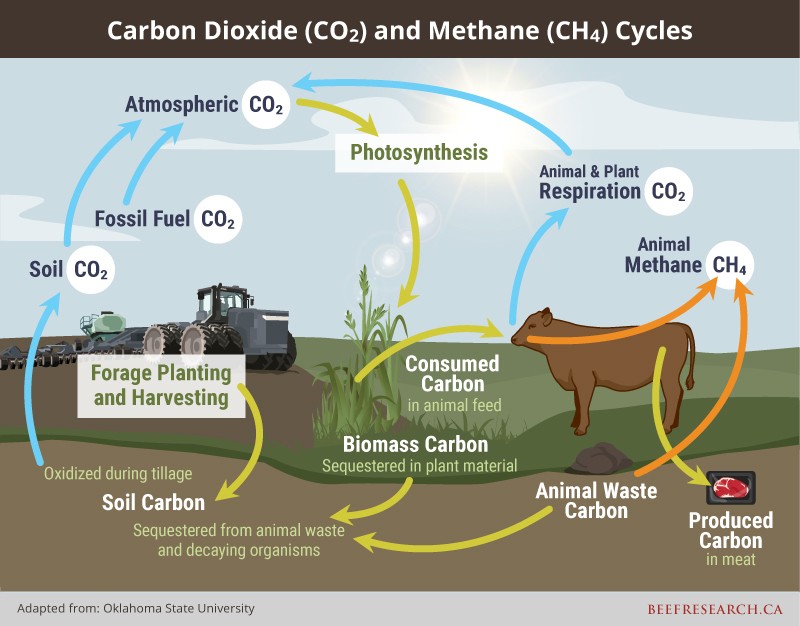
Every living thing contains carbon, and everything – including cattle, grasslands, and people – are part of the carbon cycle. Raising beef cattle can have both positive and negative impacts on the carbon cycle and different management practices can increase or decrease the sector’s carbon footprint.
Key Points |
---|
All living things contain carbon and are part of a carbon cycle. There are inputs and outputs, or “sources” and “sinks” in every carbon cycle, including beef production. |
A carbon source is a component of the cycle that emits more carbon than it sequesters. In the beef value chain, an example of a carbon source includes carbon dioxide (CO2), a greenhouse gas that is emitted when fossil fuels are used to grow, harvest, transport, or feed forage to cattle. Another example of a carbon source is enteric methane (CH4), a natural by-product of microbial fermentation in the rumen that occurs as cattle convert forage into protein. |
A carbon sink is a part of the carbon cycle that stores more new carbon than it emits over time, thereby reducing atmospheric carbon. Examples of carbon sinks include grasslands, perennial forages, forests and wetlands. |
All living things emit greenhouse gases such as carbon dioxide (CO2), methane (CH4), and nitrous oxide (N2O). Over time and due to industrialization, greenhouse gases are building up in our atmosphere and increasing the global temperature, which is referred to as global warming. |
Not all greenhouse gases have the same global warming potential (GWP). Carbon dioxide lasts in the atmosphere for hundreds of years, whereas methane, while initially having a 25-fold greater impact when it’s first emitted, has a lifespan of around 12 years.10 |
The average global temperature is expected to increase by 0.2°C per decade due to increased global human activity and industrialization.1 Over decades, increased temperatures present challenges to beef producers, such as an increased risk of extreme weather events like drought or flooding. Climate change can also lead to opportunities, such as a longer frost-free growing season and potential to grow new forages plants (such as more warm-season species) with greater yield possibilities. |
The carbon footprint for beef production varies around the world, by region, and even by farm, depending on different management practices. Measuring emissions and sequestration in beef production depends on the type of life cycle analysis performed. (i.e., birth-to-consumption vs. birth-to-slaughter). When people attempt to compare carbon footprints that analyse different portions of the beef production life cycle – or entirely different industries – these comparisons can be inaccurate or even misleading. |
Canada’s perennial forages and grasslands are critically important for carbon sequestration and storage. Scientists estimate that between 50 and 200 tonnes of carbon per hectare is stored belowground in Canada’s grasslands, with an additional 3 to 12 tonnes of carbon per hectare stored in above-ground plant growth and plant litter.4 Grasslands are more effective at protecting carbon than forests because grasslands store up to 97% of carbon belowground, unlike forests that store most of their carbon above ground. In the event of a fire, grassland carbon stores are largely unaffected while forest fires lead to high carbon loss to the atmosphere. |
There are Canadian initiatives and pilot projects underway to help producers determine how much carbon they sequester in pastures under different management systems. This may help farmers establish a value for the carbon they are sequestering and may encourage the adoption of management strategies that increase carbon sequestration. |
Farms that implement management practises that support removing carbon dioxide from the atmosphere and sequestering carbon belowground may be more resilient and adaptable to changing climatic conditions. |
Carbon Sequestration and Emissions in Beef Cattle Production
Cattle rely on rumen microbes to break down forage and produce high quality protein from plants that humans are otherwise unable to extract nutrients from. A natural by-product of rumen fermentation is enteric methane (CH4), a greenhouse gas. Incorporating different feed additives, such as monensin, can help reduce enteric methane emissions while still enabling cattle to convert roughage into nutrient-rich beef. Burning fossil fuels for feeding or forage operations is another example of carbon emissions, this time in the form of carbon dioxide (CO2). Producers can reduce carbon dioxide emissions by implementing extended grazing to reduce reliance on daily feeding.
On the other side of the beef production carbon cycle, a valuable and positive component is the use of perennial forages and grasslands, the foundation of the beef cow-calf sector. Forages, including native grasslands, are a critically important part of the carbon cycle because of their sequestration potential in Canada and worldwide. Forages and grasslands capture carbon dioxide (CO2) from the atmosphere and capture carbon above ground in plant growth, and below ground in roots. Over time, this process helps remove carbon dioxide from the atmosphere and, when occurring in soil that may be depleted in soil organic matter, can help boost soil carbon stores, offsetting a portion of the rise in atmospheric carbon.
The cattle production carbon cycle can be complex, but the basic components remain the same. The way that carbon moves through the environment will vary between different systems and production practices. For example, the carbon cycle for an extensive cow-calf beef operation will be different than that of an indoor-housed dairy due to differences in feeding strategies or housing, even though inputs (forage) and outputs (beef and milk products) may be relatively similar. Carbon cycles can also differ by region and vary around the globe. A North American production system where a cow weans a calf each year will produce less greenhouse gases per unit of output than systems in other parts of the world where a cow weans a calf every three years.
Grasslands and forage crops play a valuable role in sequestering carbon. Worldwide, grasslands store up to 30% of the world’s organic carbon.11 Plants absorb carbon dioxide (C02) from the atmosphere and store carbon in their biomass, much of which is in the root system. There are Canadian initiatives underway to help quantify carbon stores in different cattle production scenarios. This research may help demonstrate what management practices lead to better carbon storage, and more resilient agro-ecosystems.
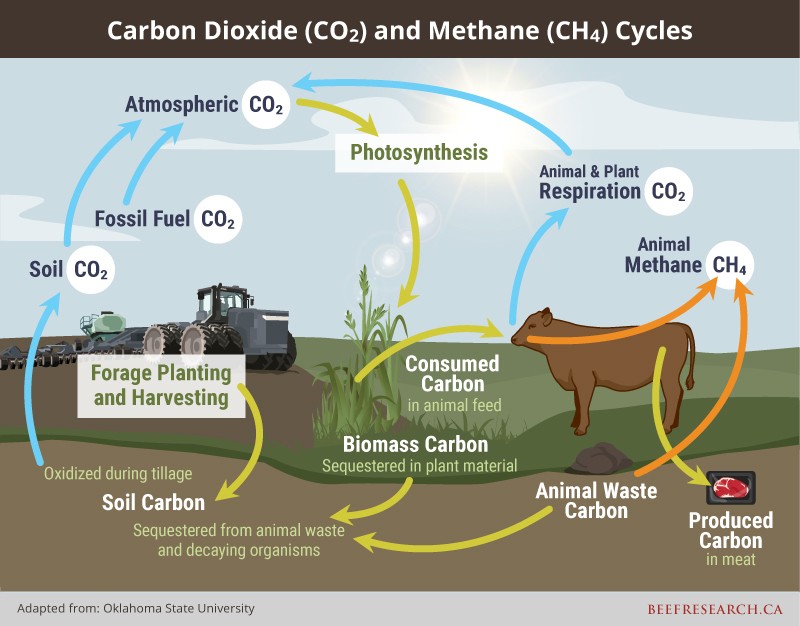
With any nutrient cycle, carbon systems have inputs and outputs which are sometimes referred to as “sources” and “sinks.”
Carbon sources are parts or processes of the system that release or emit more carbon than they absorb. In the beef value chain, this could include greenhouse gas emissions such as carbon dioxide (CO2) or methane (CH4). Burning fossil fuels in order to plant or harvest forage crops or transport beef products to retail is an example of a carbon source, as is enteric methane, emitted from cattle.
Carbon sinks are parts or processes of the system that sequester or are associated with a net increase in carbon storage. In the beef value chain, carbon sinks may include grasslands, perennial forages, wetlands, and forests.
Carbon sequestration is a term used to explain how carbon from the atmosphere, in the form of carbon dioxide (CO2), is captured by plants and stored or retained in roots, leaves, or wood over the long term.
Biogenic carbon refers to carbon that has been fixed by living plants and animals in a relatively short timeframe. For example, straw bedding, animal manure, or meat or milk would be considered biogenic carbon in a beef production system.
Inorganic carbon is part of the carbon cycle and represents the non-living carbon-containing components of the cycle. Inorganic carbon can be in the form of oxides found in the atmosphere such as carbon dioxide or carbon monoxide. Inorganic carbon is present in soils in a mineralized form, such as limestone. Other examples of inorganic carbon include allotropes like graphite and diamonds.
Grassland soils are also home to methanotrophs (bacteria and microorganisms that consume methane) and methanogens (methane-producing bacteria). On average, the combination of both lead to healthy grasslands generally being net sinks of methane, meaning grasslands are responsible for a net removal of atmospheric methane.
Greenhouse Gas Emissions
Nearly all living things, including plants and animals, produce greenhouse gases. Agriculture is estimated to be responsible for approximately 10% of global greenhouse gas emissions. Like most animals, cattle emit three different types of greenhouse gases including carbon dioxide (CO2), nitrous oxide (N2O), and methane (CH4). Methane is primarily emitted from all ruminants, including beef cattle, as enteric methane, a natural by-product of rumen microbes that ferment and break down high fibre feeds within an animal’s digestive system.
Greenhouse gases have different global warming potentials (GWP), which means not all greenhouse gases impact the global temperature equally. Methane, while initially having a stronger global warming impact than carbon dioxide for the first few years after it is released into the atmosphere, is relatively short-lived, with an atmospheric lifetime of approximately 12 years.10 Carbon dioxide, on the other hand, lasts in the atmosphere for hundreds of years and continues to accumulate.
Global Warming and a Changing Climate – Opportunities & Challenges for Beef Production
Watch the webinar A Changing Climate: is the Beef Industry Prepared?
Global human activity and industrialization has led to increased emissions of greenhouse gases, like methane (CH4) and carbon dioxide (CO2) into the atmosphere. The global temperature has increased by 0.6°Celsius in the past hundred years, which has been attributed to this increase in greenhouse gases. Average global temperatures are estimated to increase by 0.2°C per decade for the next two decades, which present both challenges and opportunities for agriculture.1
It’s thought that increased global temperatures will cause more frequent severe weather events, such as droughts and floods, as well as an increase in evaporation from soils, a decrease in water availability for plants, and a reduction in stock water supplies. There is also a projected risk of increased wildland fires, which can negatively affect growing conditions and reduce opportunities for grazing. The ability of beef producers to adapt to changes and create resilient, stable farming environments that are able to withstand variable conditions will be important for Canada’s beef sector.
A warming climate also creates potential opportunities, such as a longer frost-free growing season for many regions which may lead to the ability to grow different crops with potentially greater yields, including warm season species such as corn, sorghum, and millet. As well, warmer winter temperatures may improve winter feed efficiency.
Other potential climate-related challenges producers should consider include:
- Increased occurrence of freeze and thaw cycles which can affect animals during winter grazing.
- As temperatures increase, there may be an increase in parasites and diseases carried by insects as the distribution and habitat of pests increases.
- Increased risk of heat stress and cold stress on traditional Canadian cattle breeds.
- Supply chain vulnerabilities due to weather-related disruptions that could impact processing, refrigeration, and transport.
- Increased insurance claims and associated premiums due to crop insurance and catastrophic weather events.
While there are uncertainties about the future, Canada’s beef sector is already working to improve their environmental footprint and address challenges, and has made notable improvements in the past several decades. An analysis of Canada’s beef production from 1981 to 2011 demonstrated that Canada produced 32% more beef in 2011 while emitting 15% fewer greenhouse gases. In addition to reducing greenhouse gas emissions, the analysis also found that producing the same amount of beef in 2011 required 29% less breeding stock, 27% fewer slaughter cattle, 24% less land, and used 17% less water than in 1981. Innovation and technology, applied in the lab and on the farm, will enable Canada’s beef farmers to continue to improve the sector’s environmental track record.
Comparison Confusion
Some industries, such as transport, have been measuring carbon footprints for decades. In beef cattle production, the practice of measuring emissions and sequestration is a relatively new field of study. The way that carbon is analysed depends on the type of life cycle analysis that is performed. The interrelationships between crops and livestock as well as the diversity of production systems across Canada make developing a single approach for beef life cycle analysis challenging.
If the entire beef value chain isbeing evaluated in a birth-to-consumption analysis, all inputs and outputs are accounted for from birth through feeding, slaughter, retail, and finally consumption. Other types of analyses focus on a portion of the beef cycle, such as birth-to-slaughter. For this type of analysis, the carbon footprint of beef cattle and all related forage, fuel, irrigation, feeding, finishing, and transport inputs and outputs are estimated.
When people attempt to compare results from carbon footprint studies that analyse different portions of the beef production cycle, comparisons are inaccurate and even misleading. It becomes even more challenging to compare the carbon footprints of different industries (i.e., air travel vs beef cattle) or between different agricultural sectors.
Carbon Sequestration on Grasslands
Perennial forages and grasslands are the foundation of the cow-calf sector and are a critically important part of the carbon cycle because of their sequestration potential in Canada as well as globally. Grasslands store up to 30% of the world’s organic carbon, and perennial grasslands are particularly effective carbon sinks, storing up to 97% of their carbon belowground.4
All plants, including trees, annuals, and native and tame perennial grasslands, sequester carbon, however not all carbon sinks are equal. In stable conditions, forests will sequester more carbon in timber, leaves and needles, than grasslands store above and below ground. However, when a forest fire occurs, much of this carbon is promptly released back to the atmosphere during combustion. While forested rangelands are important ecosystems for many land uses including beef cattle production, fire suppression, drought and insect infestations may create greater forest fire risk, thereby putting this carbon at risk of release. In contrast, by storing the majority of their carbon belowground where it is protected from fire, grasslands provide a more stable carbon sink.
Annual crops sequester the least amount of carbon underground. Annual cropping systems are important for food and feed production, however compared to native grasslands, annual cropland often provides fewer ecosystem benefits, such as reduced biodiversity, reduced carbon storage, decreased soil water availability, and decreased soil porosity.3
Scientists estimate that between 50 and 200 tonnes of carbon per hectare is stored below ground in Canada’s grasslands, with an additional 3 to 12 tonnes of carbon per hectare captured in above-ground plant growth and plant litter.4 Most of the carbon sequestered is typically contained in the top 15cm of the soil. Soil organic carbon can also increase with grazing, particularly in the surface (0-15cm) soil, with minimal changes below this depth.6 Increased soil carbon due to grazing is caused by the ongoing animal impact that is responsible for trampling litter into soil, a grazing-induced plant response which causes plants to set down additional shallow roots following grazing, as well as an increase in the growth of individual plant species with higher grazing tolerance.6
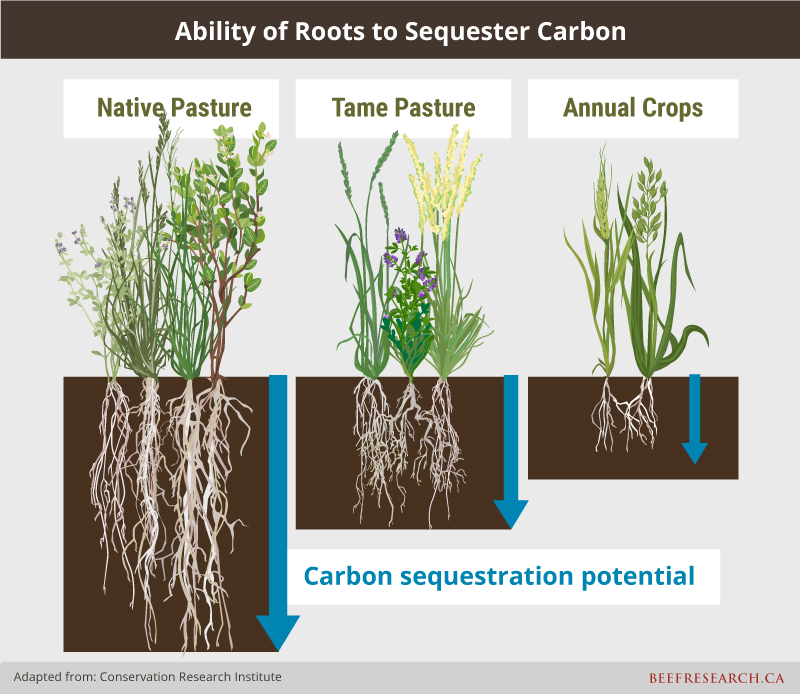
Carbon sequestration is a dynamic process. Plants take in carbon dioxide (CO2) from the atmosphere during photosynthesis for plant growth, release oxygen into the atmosphere, and sequester carbon underground within their root systems. Following root death this leads to carbon addition to the mineral soil.
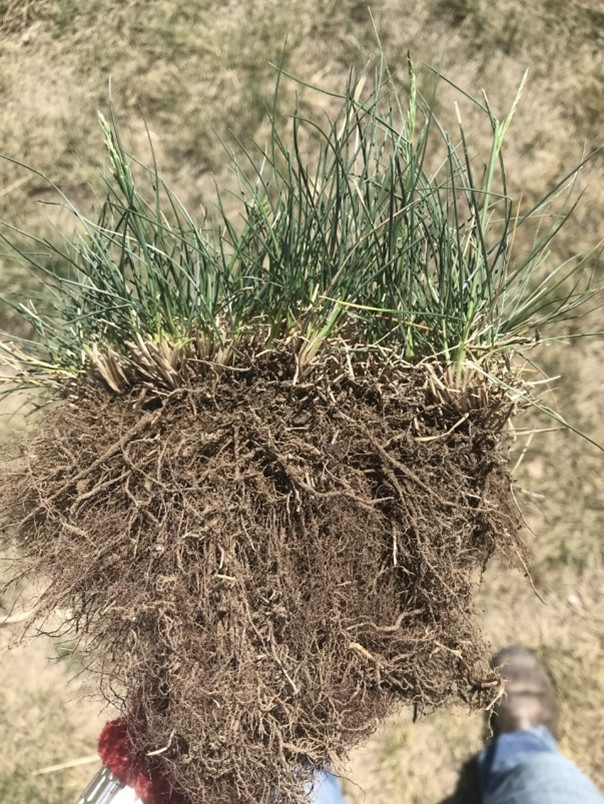
Carbon fluxes throughout the year within a grassland ecosystem in North America, with intense periods of carbon uptake during plant growth in the spring and summer months. This uptake tapers off in late summer and fall and is followed by weak carbon losses as plant litter is broken down by soil microbes.3,9 When carbon inputs from plant growth are greater than the decomposition of existing soil carbon throughout the year, there is a net removal of CO2 from the atmosphere and grassland soil organic carbon increases. When decomposition rates match vegetation growth, the system is in equilibrium, and when decomposition rates are greater than plant growth, there is a net carbon loss from the system.10 Precipitation, temperature, soil zone, and even plant species all play a role in grassland carbon dynamics. For example, increased moisture will quicken decomposition and the loss of soil organic carbon, but those losses are often offset by increased plant growth.9
Measuring how much carbon is stored in Canadian grasslands is a challenge and a gap that scientists are actively working to address. Soil organic carbon can be readily measured at a single point in time, however carbon increases (or decreases) over time that are related to aboveground practices like grazing are challenging to pinpoint, in part due to the small magnitude of changes that may occur over decades.
Carbon Storage and Land Use Practices
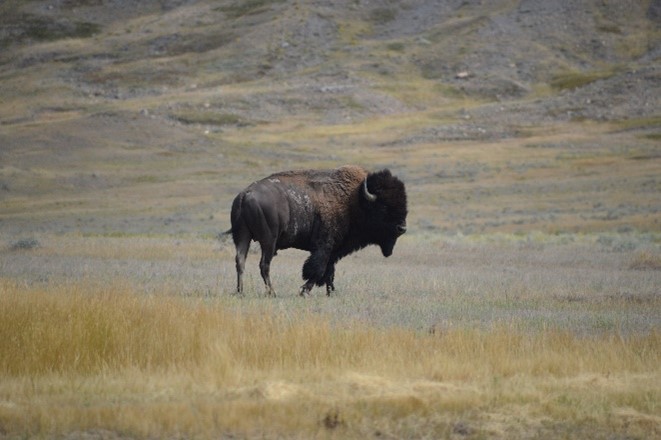
North American grasslands evolved under bison grazing and prairie fire, and scientists suggest the carbon cycle was largely self-regulated in this environment. Settlement and land conversion started to take place in the 1900’s, and major environmental disruptions, including the severe drought and dust storms of the 1930’s (i.e., the Dust Bowl era), demonstrated that prairie grasslands were vulnerable to management decisions.
Land use change is the single largest disturbance of grassland carbon, leading to an estimated global loss of 30-50% of grassland carbon stores.4 Practices such as converting grassland to cropland, draining wetlands, and construction such as urban development and transport infrastructure, lead to carbon loss. Changes in carbon stocks occur in the short term, such as when land use change first occurs, however long-term carbon loss may continue.10 For example, when a grassland is cultivated for annual cropping, there will be a rapid loss in carbon stocks during the first few years the land is cultivated, however carbon may continue to be lost from the system for several years after, eventually reaching an equilibrium at a much lower level. Beneficial land use practices, such as revegetating annual cropland to perennial forage or using livestock manure as a fertilizer can help rebuild carbon stocks, but it remains unclear as to whether the previous carbon stocks can be restored, and if so, how long it may take.3
It’s estimated that Canada once had 61,500,000 hectares of natural grassland, of which approximately 1,540,000 hectares remain, primarily in smaller fragments. The conversion of natural grassland to annual cropland represents a loss of carbon stocks estimated between 20% and 60%.11 While grasslands sequester carbon and cycle other nutrients, these valuable landscapes also provide numerous ecological services including biodiversity conservation; habitat for wildlife, species at risk, and pollinators; flood mitigation; sediment trapping and buffering; water filtration and retention; and soil conservation. The conversion of grasslands to other land uses impacts carbon sequestration negatively, but also causes a loss of other ecological benefits.
Left intact, Canadian grasslands can and have been building carbon stores. In a study that looked at carbon sequestration over an 80-year time frame, there was a general gain in net carbon on grazed pastures in the Great Plains, particularly in recent decades, and soil organic carbon increased during that time frame at a rate of 190 kg of carbon per hectare per year.4,10,11 The amount of estimated carbon gains varied by soil type and plant species composition, with black soils sequestering more carbon than dark brown and brown soils, respectively, and fescue grassland sequestering more carbon than mixed grass and dry mixed grass ecotypes. Sequestration can also plateau and level off under stable management.
Management Practices to Increase Carbon Sequestration
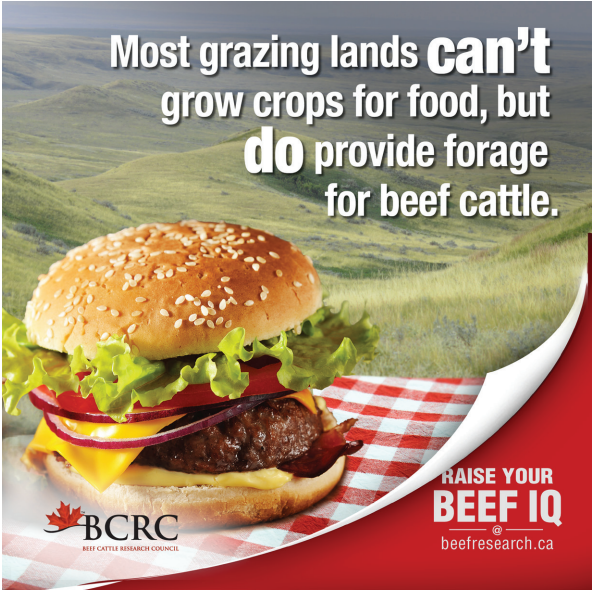
Farms that implement management practices that promote removing carbon dioxide from the atmosphere (through plant growth) and sequestering that carbon belowground (in the root system and soil) may be more resilient and adaptable to changing climatic conditions. There are some on-farm practices that producers can implement to increase carbon sequestration and stabilize their agricultural landscapes:
- The best way to maintain or increase carbon sequestration is to avoid converting perennial grassland to annual cropland. After annual cropland is planted to perennial forage, revegetated land is slow to recover and may not fully recover root mass and soil organic matter, even after 50 years.3
- Converting annual cropland to perennial forage is a carbon storage opportunity, however data is unclear on how much carbon is sequestered over time. What is clear is that it takes a long time for carbon to build up, making short-term carbon sequestration measurement a challenge. In addition to carbon storage, converting annual cropland, particularly marginal land, to perennial forage does provide many additional ecological benefits, including improved habitat and biodiversity, reduced soil erosion, and increased water retention.
- Canadian studies have shown that moderate grazing can increase soil carbon concentration, particularly in the top 15cm of the soil surface.6 However, scientists have learned that environmental aspects, such as moisture conditions, tend to be the driving factor for soil carbon stores by regulating plant growth (i.e., carbon inputs). Grazing appears to be more beneficial for soil carbon in moister grasslands than in dry areas. Moderate grazing can also enhance other ecological services, including plant diversity and forage production.
- Native grassland plant species tend to have higher root-to-shoot ratios than tame forages and tend to have more root mass belowground than tame species.3 When revegetating annual cropland to perennial grassland, including native plant species may be an effective way to increase carbon storage.
Wetlands and Carbon
Wetlands, similar to grasslands, provide many ecological services including flood mitigation, biodiversity conservation, energy and nutrient dissipation, forage, and habitat. Wetlands are valuable carbon sinks, however because of increased bacterial activity and decomposition, they are also potential sources of greenhouse gas emissions.
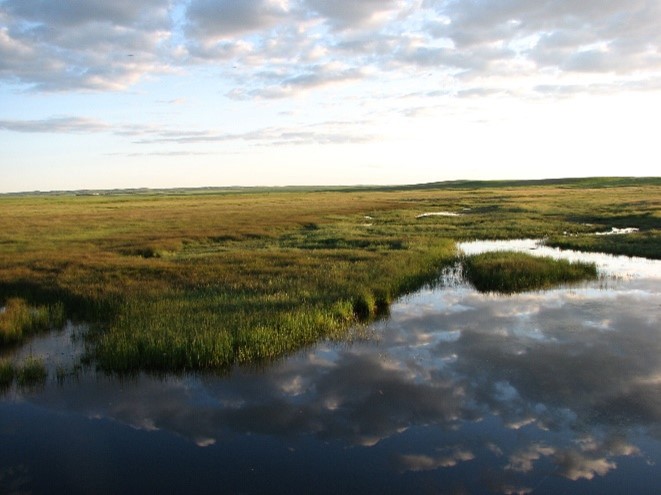
Left undisturbed, wetlands typically store more carbon than they release, resulting in net carbon sequestration. However, wetland habitat is often modified by ditching, tile draining, or other practices in order to allow cropping on land that would otherwise be too wet to support annual crops. These practices are regulated by region and province and are often controversial.2
Wetland restoration can help re-establish ecological functions on these sites, and Canadian studies have indicated that carbon sequestered on newly restored wetlands as well as older restored wetlands result in a net carbon benefit.7
Carbon Offsets
There is a growing interest in trading carbon through credits or offsets. Industries or companies that may be required to or volunteer to offset their greenhouse gas emissions may look to purchase credits or offsets from organizations that can quantify carbon stores from perennial grasslands. Pilot projects across Canada are being set up, including the Canada Grassland Project Protocol. Led by the Canadian Forage and Grassland Association, this project, which has numerous provincial and national partners, will help producers determine how much carbon they sequester in pastures and wetlands under different management practices and production systems. When carbon stores are better quantified on a farm-level, farmers may be able to establish a value for the carbon they are sequestering and trade carbon offsets in the future.
Moving Forward
There is much to learn and more work to do, however Canadian researchers are working toward understanding practices that support carbon sequestration and methane reduction in beef cattle production. At the same time, technology and innovation are allowing producers to become more efficient at producing beef.
- Scientists understand that converting native prairie to cropland results in a loss of carbon stored in the ground, however less is known about how different land uses, such as converting annual cropland to perennial forage, impact carbon storage over time. Learn more about current research on carbon storage predictions.
- Measuring how much carbon is stored by Canadian wetlands is an existing gap that that scientists are working to address.
- Grazing different legume species can affect methane emissions. Recent work indicates that cattle grazing cicer milkvetch resulted in decreased methane production compared to cattle grazing the control pasture blend. This warrants further study.
- Increasing the fat content in legumes is a potential method to reduce methane emissions from grazing cattle. A current project is looking at how to enhance total lipid content in alfalfa and sainfoin.
- Adding legumes to grassland pasture mixes can increase carbon stores. Ongoing work in eastern Canada is assessing how to incorporate legumes and maintain them in pasture stands.
- Feed additives, such as ionophores like monensin, incorporated into feedlot rations can help to reduce methane production.
- Effective manure management can increase organic matter and carbon on pastures and cropland.
Beef production in Canada has a distinct carbon cycle that includes inputs and outputs, and greenhouse gas emissions and carbon sequestration. While there are challenges that the sector must overcome, including reducing emissions and meeting ambitious industry-leading targets, there is also a lot of potential. Improved practices are allowing producers to be more efficient at producing beef and there is an opportunity for a greater understanding of the incredible value that grasslands and forages present for carbon sequestration and numerous other ecosystem services.
The Bigger Picture
Learn more about the environmental footprint of beef in Canada.
Acknowledgements
Thanks to Dr. Edward Bork, Mattheis Chair at the University of Alberta, and Dr. Tim McAllister, Agriculture and Agri-Food Canada, for contributing their time and expertise to reviewing this page.
- References
-
1. Agriculture and Agri-Food Canada. 2020. Climate Scenarios for Agriculture. Available here.
2. Badiou, P., McDougal, R., Pennock, D., and Clark, B. 2011. Greenhouse gas emissions and carbon sequestration potential in restored wetlands of the Canadian prairie pothole region. Wetlands Ecology and Management. 19: 237-256. Available here.
3. Bork, E. 2019. Grassland Carbon: a review of current research efforts in support of biological carbon storage in Canada. University of Alberta. Presentation made to Biological Carbon Canada.
4. Bork, E. and Badiou, P. 2017. The importance of temperate grasslands in the global carbon cycle. University of Alberta and Ducks Unlimited Canada. Available here.
5. Bremer, E. 2008. Potential of rangelands to sequester carbon in Alberta. Prepared for Alberta Sustainable Resource Development. Available here.
6. Hewins, D.B., Lyseng, M.P., Schoderbek, D.F., Alexander, M., Willms, W.D., Carlyle, C.N., Chang, S.X., and Bork, E.W. 2018. Grazing and climate effects on soil organic carbon concentration and particle-size association in norther grasslands. 8:1336. Nature.com/scientificreports. Available here.
7. McAllister, T.A., 2020. AB NAWMP Peer Review: Critical Review of Five Carbon Reports: An assessment of validity, technical merit, timeliness, relevance and gaps. Alfalfa Seed Committee. Available here.
8. OMAFRA. 2013. Beef Cattle and Greenhouse Gas Production. Available here.
9. Sanderson, J.S., Beutler, C., Brown, J.R., Burke, I., Chapman, T., Conant, R.T., Derner, J.D., Easter, M., Fuhlendorf, S.D., Grissom, G., Herrick, J.E., Liptzin, D., Morgan, J.A., Murph, R., Pague, C., Rangwala, I., Ray, D., Rondeau, R., Schulz, T., and Sullivan, T., 2020. Cattle, conservation, and carbon in the western Great Plains. Journal of Soil and Water Conservation. 75 (1) 5A-12A. Available here.
10. Viresco Solutions Inc. 2019. Global Assessment of Beef Emissions Quantification Standards and Tools. Global Roundtable for Sustainable Beef (GRSB) Phase I Report
11. Wang, X. VandenBygaart, A.J., and McConkey, B.C. 2013. Land Management History of Canadian Grasslands and the Impact on Soil Carbon Storage. Rangeland Ecology & Management, 67(4):333-343. 2014. Available here.
Feedback
Feedback and questions on the content of this page are welcome. Please e-mail us.
Expert Review
This content was last reviewed June 2021.
This topic was last revised on December 11, 2023 at 9:49 am.